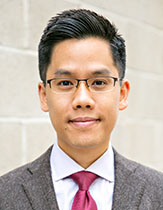
The technology behind dual-energy CT (DECT) has been around for nearly 4 decades1,2, but it was not until 2006 that the first dual-source CT scanner became available for clinical use.3 The years that followed brought about further technologic advances, including the development of additional techniques for obtaining DECT data. Modern DECT imaging can be performed with radiation doses comparable to conventional single-energy CT (SECT).4 Applications of DECT exist throughout the human body, with many important applications in neuroradiology. Familiarity with the principles, applications, and potential pitfalls of DECT will be important as the use of clinical DECT becomes increasingly common.
The relationship between the CT attenuation of different materials and photon energy forms the basis of DECT.5 The CT number of a voxel is related to its linear attenuation coefficient. The linear attenuation coefficient is not only a function of material composition, but also a function of material mass density and the photon energies interacting with the material.6 Notably, linear attenuation coefficients of elements with higher atomic numbers, such as iodine and calcium, have a greater energy dependence than those of elements with low atomic numbers, such as hydrogen, carbon, nitrogen, and oxygen, which make up the bulk of the human body.5 Under certain conditions, different materials can exhibit similar attenuation on SECT. In DECT, however, attenuation measurements are obtained at 2 different energy spectra, allowing for such materials to be distinguished from one another provided that they differ sufficiently in atomic number.5 DECT data can be postprocessed with material decomposition algorithms to generate quantitative iodine maps , virtual noncontrast (VNC) images, and virtual noncalcium (VNCa) images.7 Additionally, simulated SECT images can be generated, including weighted average images created by blending low- and high-energy data, and virtual monochromatic images (VMIs).4
Perhaps the most widely recognized advantage of DECT over SECT in neuroradiology is its ability to distinguish intracranial hemorrhage from iodinated contrast. DECT is highly accurate in differentiating hemorrhage from contrast throughout the intracranial compartment.8 In patients with acute ischemic stroke who have undergone mechanical thrombectomy, DECT can aid in the early distinction between hemorrhage and contrast.9 DECT could have a growing role in the evaluation of postthrombectomy patients at comprehensive stroke centers with the increase in thrombectomy utilization for acute ischemic stroke, following the results of the recent DAWN10 and DEFUSE-311 trials.
DECT is also useful in differentiating tumor-related intracranial hemorrhage from pure intracranial hemorrhage.12 The feasibility of radiation dose reduction using VNC images obtained from DECT angiography data in the evaluation of subarachnoid hemorrhage was recently investigated.13
Other important DECT applications include metal artifact reduction in the evaluation of treated intracranial aneurysms14,15, metal artifact reduction in the instrumented spine using VMI16–18, bone subtraction for extra-axial hemorrhage detection19,20, and bone and calcium subtraction for cerebral aneurysm21 and carotid stenosis evaluation22, respectively. DECT has also been applied to head and neck imaging to improve soft tissue characterization23, as well as bone marrow imaging in multiple myeloma24 and vertebral compression fractures25 using a VNCa technique.
In this installment of the AJNR News Digest, some of the more recent publications in AJNR on DECT are highlighted. One shows the ability of DECT to function not only as a diagnostic tool, but also as a potential predictor of hemorrhagic complications in postthrombectomy patients.26 Another shows that DECT may aid in the detection of residual thromboembolic material or early rethrombosis shortly after thrombectomy, without the need for additional contrast administration.27 Two articles reveal potential pitfalls in the interpretation of cerebral contusions28 and subdural effusions29 that can be avoided with DECT. Finally, a quantitative study defining the optimal DECT virtual monochromatic images for the evaluation of normal head and neck tissue and head and neck squamous carcinoma is revisited.30