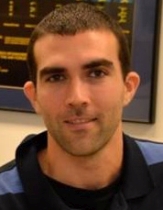
Greg Byrne
Computational fluid dynamic simulations using patient-specific vascular geometries provide a powerful tool for understanding, treating, and preventing a variety of cerebrovascular diseases. The underlying goal of our research is to use such patient-specific modeling to connect hemodynamic variables with clinical events, thereby gaining a better understanding of intracranial aneurysm development and the mechanisms that may be responsible for their rupture. Hemodynamic variables have the potential to provide important quantitative information that can be used to improve clinical risk assessments, which, at the moment, rely largely on the use of a few image-based metrics derived from the aneurysm geometry (size, aspect ratio, etc).
Our study used 2 methods of analysis designed to provide an objective and quantitative characterization for the large-scale spatiotemporal properties of intracranial blood flows. These methods provide 2 quantities that allow us to connect specific large-scale hemodynamic structure to rupture. The first method of analysis targeted coherent swirling flow structures (vortices) that are commonly formed within the aneurysm sac as a result of recirculating blood flow. We identified these vortices by constructing 1-dimensional vortex axes known as “vortex core lines,” around which the flow rotates. These core lines provide a useful tool for visualizing the skeletal structure of the blood flow and, by estimating their total length, they also provide a simple quantitative measure to characterize the spatial flow complexity. The second method of analysis used proper orthogonal decomposition to separate the spatial and temporal dynamics of the blood flow into a weighted sum of the dominant (most energetic) spatial flow structures. Using this decomposition, we were able to estimate an entropy measure for the flow over the cardiac cycle by computing how the energy is distributed among the dominant spatial flow structures. This provides a simple quantitative measure to characterize temporal flow stability.