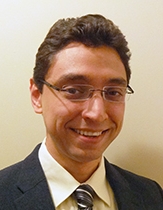
Guest Editor
Carlos Zamora
Susceptibility-weighted imaging (SWI) is a high-resolution, fully velocity-compensated gradient-echo MRI sequence that maximizes intrinsic contrast differences between tissues by virtue of their local magnetic properties. This technique exploits susceptibility information contained within phase acquisitions and merges such with magnitude data to generate a clinically usable set of images. Notably, the functional incorporation of phase data in a clinical sequence was initially challenging and warranted improvements in MRI technology that eventually culminated in the development of SWI. The entire sequence requires no more than a few minutes of scanning time and can be effectively applied in a clinical setting.
SWI has proven to be highly sensitive for the detection of paramagnetic and diamagnetic substances such as hemosiderin, ferritin, calcium, and deoxyhemoglobin, which has resulted in a number of research and clinical applications being advocated. There has also been a growing interest in taking advantage of the enhanced sensitivity and resolution provided by ultra-high-field scanners for both functional and microstructural applications.1–3 Over the past several years, SWI has gained relatively wide clinical acceptance, and many potential diverse uses have been proposed in the literature, including in trauma,4 aging and neurodegenerative disorders,1,5–7 demyelination,8 and stroke,9–11 among others. In general, at least in the clinical setting, SWI seems to be most popular by virtue of its exquisite sensitivity for the detection of small amounts of blood products that may otherwise be subtle, or even invisible. However, in addition to structural abnormalities, hemodynamic information can be derived visually and with techniques such as quantitative susceptibility mapping, which can provide measures of oxygen extraction.12,13
SWI was originally introduced as a means for intrinsic MR venography based on signal changes in response to deoxyhemoglobin.14 Studies have shown that SWI can provide good visual representation of cerebral veins and new techniques may even be able to consistently remove air/tissue interface artifacts in the future. However, beyond the demonstration of venous morphology, relative changes in signal intensity provide an additional level of functional information by reflecting oxygen metabolism. Deoxyhemoglobin exerts a strong influence on SWI signal due to its paramagnetic properties, and this feature is exploited as a marker of cerebral hemodynamics. Cortical draining veins will vary their signal as a function of perfusion status and variations in oxygen extraction, wherein draining veins in hypoperfused tissue will have lower signal and artifactually appear more prominent, and vice versa. As the use of SWI has become more generalized, my own awareness of this additional layer of hemodynamic information has also increased. For instance, aside from expected changes in cases of stroke, I more frequently see patients where perfusion abnormalities were present and sometimes unsuspected, which may be particularly helpful when the initial clinical picture is not entirely clear. Examples of such cases may include postictal states, migraines, and various causes of vasospasm.
While an attractive feature of SWI is the ability to visually derive hemodynamic information, many studies have proposed quantification of signal changes, which may make analysis more reproducible. A similar sequence, susceptibility-weighted angiography (SWAN, based on magnitude but not phase information) was able to differentiate normoxemic from hypoxemic veins both visually and through quantification of voxel intensities.15 Such properties are particularly appealing in the evaluation of stroke. In fact, blood oxygen level-dependent sequences, including SWI, have been proposed as an alternative (and possibly more accurate) means for determining tissue at risk based on oxygen metabolism.16-18 If these uses can be clinically validated, they would provide a significant added benefit by not requiring intravenous contrast material. They also have much higher spatial resolution than arterial spin-labeling, the only noncontrast MRI perfusion sequence in routine clinical use today.
The current issue of AJNR Digest focuses on the added value of SWI as a marker of cerebrovascular hemodynamics and vasomotor reactivity. The first two review articles come from the same group that developed SWI.19,20 In part 1, they carefully expose the technical aspects on which the sequence is based, recommend imaging parameters for different field strengths, and examine concepts that are essential for optimal clinical interpretation.19 Part 2 explores various clinical applications, including the evaluation of cerebrovascular and perfusion abnormalities.20
The paper by Chang et al21 highlights the sensitivity of SWI to venous oxygenation by demonstrating visual and quantifiable perfusion changes in healthy volunteers during apnea and hyperventilation, in response to CO2 as a vasoactive modulator.